Electron-rich and electron-deficient impurities are distinct in their influence on material properties. Electron-rich impurities, like phosphorus in silicon, introduce extra electrons, enhancing conductivity and modifying the material’s band structure. They bolster the number of negative charge carriers, crucial in technologies like solar cells and LEDs.
Conversely, electron-deficient impurities, such as boron in silicon, create “holes” or vacancies, elevating conductivity by facilitating the flow of positive charge carriers. These impurities modify band structure, impacting electronic behavior and finding applications in transistors and semiconductor devices.
Both impurity types play pivotal roles in semiconductor engineering, with their controlled incorporation driving innovations in electronics, offering a spectrum of functionalities essential for diverse technological applications.
What is Electron-Rich Impurities?
Electron-rich impurities are atoms or molecules that introduce excess electrons into a material’s structure, altering its electrical properties. These impurities possess more electrons in their outer shells than the host material, causing a surplus of negatively charged particles within the material lattice.
When added to a semiconductor, for instance, electron-rich impurities, such as phosphorus or arsenic in silicon, introduce extra electrons into the crystal lattice. These additional electrons become mobile charge carriers, enhancing the material’s conductivity. The surplus electrons occupy energy levels within the bandgap, influencing the material’s electrical behavior.
Moreover, electron-rich impurities modify the semiconductor’s band structure, affecting its optical properties by enabling the absorption and emission of specific wavelengths of light. This phenomenon finds applications in technologies like photovoltaic cells and light-emitting diodes (LEDs).
Controlling and introducing electron-rich impurities are pivotal in semiconductor manufacturing. Techniques like doping during crystal growth or ion implantation allow precise control over impurity concentrations, crucial for tailoring material properties to meet specific technological needs.
Electron-rich impurities play a significant role in enhancing conductivity, modifying electronic behavior, and enabling numerous semiconductor applications, making them essential elements in modern electronics and optoelectronic devices.
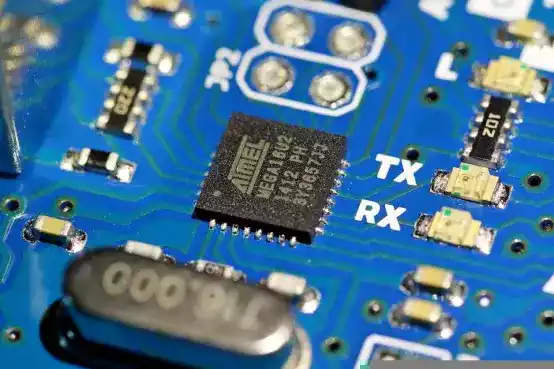
What are Electron-Deficient Impurities?
Electron-deficient impurities refer to atoms or molecules that possess fewer electrons in their outer shells compared to the atoms in the host material. When incorporated into a material’s lattice, these impurities create electron deficiencies or “holes” within the crystal structure.
For example, in semiconductor materials like silicon, electron-deficient impurities such as boron or gallium have fewer electrons in their outermost shell than silicon. When these impurities are introduced into the crystal lattice, they create vacancies where electrons would normally reside. These vacancies act as positive charge carriers or “holes” within the material, contributing to its conductivity.
Electron-deficient impurities modify the material’s band structure, affecting its energy levels and electrical behavior. They influence the movement of charge carriers, impacting the conductivity and electronic properties of the material. In electronic devices, these holes facilitate the flow of positive charge, contributing to the functionality of transistors, diodes, and other semiconductor components.
The controlled introduction of electron-deficient impurities, achieved through doping techniques during fabrication processes, is crucial in the semiconductor industry. These methods enable precise manipulation of impurity concentrations to tailor the material’s properties for specific applications.
In summary, electron-deficient impurities play a fundamental role in altering the conductivity, band structure, and electronic behavior of materials, making them indispensable in the design and functionality of various semiconductor devices and technologies.
Comparison chart of Electron Rich and Electron Deficient Impurities
Here’s a comparison chart highlighting the key differences between electron-rich and electron-deficient impurities:
Characteristics | Electron-Rich Impurities | Electron-Deficient Impurities |
---|---|---|
Electron Configuration | Contain extra electrons compared to host material | Possess fewer electrons than the host material |
Effect on Material Conductivity | Enhances conductivity by introducing extra electrons | Increases conductivity by creating “holes” or vacancies |
Band Structure Modification | Occupies energy levels within the bandgap, altering bandstructure | Modifies band structure by creating holes, affecting energy levels |
Charge Carrier Influence | Increases the number of negative charge carriers (electrons) | Generates positive charge carriers (holes) |
Impact on Electronic Behavior | Alters electrical properties by providing excess electrons | Affects electrical behavior by creating vacancies for electrons |
Examples | Phosphorus or arsenic in silicon | Boron or gallium in silicon |
Applications | Used in devices like solar cells, LEDs, and transistors | Employed in transistors, diodes, and semiconductor technologies |
Doping Techniques | Utilizes doping methods for controlled introduction | Requires doping techniques for controlled incorporation |
Importance of impurities in altering material properties
Impurities play a crucial role in altering material properties, imparting unique characteristics that are often essential for various applications.
Here’s a concise explanation of their significance:
The introduction of impurities into materials is not merely contamination but rather a deliberate process crucial for tailoring and enhancing specific properties. These impurities modify the electronic structure, conductivity, and mechanical properties of materials, enabling a wide range of functionalities.
Controlling Electrical Conductivity: Impurities, such as dopants in semiconductors, significantly impact electrical conductivity. Electron-rich impurities introduce extra charge carriers, enhancing conductivity, while electron-deficient impurities create holes, also influencing conductivity. This control over conductivity is vital in electronics, enabling the development of transistors, diodes, and other semiconductor devices.
Modifying Mechanical Properties: Impurities alter the mechanical strength, hardness, and ductility of materials. Even in small concentrations, impurities can significantly affect a material’s structural integrity, making them valuable in strengthening alloys or modifying material flexibility as needed in various industries, including automotive and construction.
Tuning Optical Properties: Certain impurities modify a material’s optical behavior. For instance, in semiconductor-based technologies, impurities influence bandgap energies, enabling the development of materials with tailored optical properties for lasers, LEDs, and photovoltaic devices.
Enhancing Specific Characteristics: Impurities can imbue materials with specific properties like magnetism, corrosion resistance, or catalytic activity. For instance, introducing impurities into steel alters its magnetic properties, allowing its application in diverse fields from electronics to construction.
Customizing Material Functionality: By strategically introducing impurities, material scientists can fine-tune a material’s properties to suit specific industrial, technological, or research needs. This customization is pivotal in advancing fields like nanotechnology, where precise control over material properties is critical.
Understanding and manipulating impurities in materials is essential for tailoring properties to meet various application demands across multiple industries. Their deliberate incorporation and control empower material scientists and engineers to design and develop materials with diverse and enhanced functionalities, paving the way for innovation in numerous technological domains.
Techniques for Detecting Electron-Rich and Electron-Deficient Impurities
Detecting electron-rich and electron-deficient impurities in materials involves specialized techniques aimed at identifying alterations in the electronic structure and conductivity.
Here are some methods for their detection:
Hall Effect Measurement: This method is used to identify the type and concentration of impurities in semiconductors by measuring the Hall voltage produced when a magnetic field is applied perpendicular to the current flow. It helps distinguish between electron-rich and electron-deficient impurities based on the sign of the Hall voltage.
Electrical Characterization: Conductivity measurements provide insights into the type and density of impurities present in a material. Electron-rich impurities increase the material’s conductivity, while electron-deficient impurities lead to a rise in resistivity.
Spectroscopic Techniques: Techniques like photoluminescence spectroscopy and X-ray photoelectron spectroscopy (XPS) analyze the material’s optical and electronic properties. They can identify impurities by studying shifts in energy levels or spectral lines corresponding to the presence of excess or deficient electrons.
Secondary Ion Mass Spectrometry (SIMS): This technique detects impurities by bombarding the material’s surface with high-energy ions, causing the release of secondary ions. By analyzing these ions’ mass-to-charge ratios, SIMS identifies the elemental composition and concentration of impurities.
Scanning Tunneling Microscopy (STM): STM images surface at atomic scales, revealing local variations in electronic structure caused by impurities. It can identify individual impurity atoms and their impact on the material’s electronic properties.
Transmission Electron Microscopy (TEM): TEM allows visualization of atomic structures and defects, helping in identifying impurities and their distribution within a material.
Each technique offers a unique approach to detect and characterize electron-rich and electron-deficient impurities, providing crucial insights into material properties and aiding in the development of advanced electronic devices and semiconductor technologies.
Real-world Applications and Significance
Impurities in materials play a pivotal role in a multitude of real-world applications, showcasing their significance in various industries:
Semiconductor Electronics: In semiconductor manufacturing, controlled introduction of impurities—doping—enables the creation of p-n junctions, essential for diodes and transistors. Electron-rich and electron-deficient impurities determine a semiconductor’s conductivity, influencing the functionality of devices like microchips, solar cells, and LEDs.
Metallurgy and Alloy Development: Impurities are deliberately added to metals to alter their mechanical properties. Alloying elements introduce impurities that enhance strength, hardness, and resistance to corrosion, crucial in constructing durable materials for automotive, aerospace, and structural applications.
Medical Imaging and Nuclear Technology: Impurities, such as radioactive isotopes, find application in medical imaging and treatment. Radioactive impurities, when incorporated into pharmaceuticals or tracers, aid in diagnostic imaging and cancer therapy.
Catalysis and Chemical Industry: Impurities in catalysts facilitate chemical reactions, accelerating or controlling the production of various compounds. They are vital in the manufacture of plastics, fertilizers, and pharmaceuticals, enabling efficient and selective reactions.
Optoelectronics and Photonics: Impurities in optical materials dictate their light-emitting or light-absorbing properties. This is exploited in fiber optics, telecommunications, lasers, and photovoltaic devices, where impurity-controlled materials drive advancements in data transmission and renewable energy.
Environmental and Energy Applications: Impurities are integral in developing materials for clean energy technologies. For instance, impurity-tailored materials in fuel cells, batteries, and energy storage systems contribute to efficient energy conversion and storage, impacting sustainable energy solutions.
Nanotechnology and Advanced Materials: Impurity engineering is crucial in nanomaterials, allowing precise control over properties like size, conductivity, and surface characteristics. This influences advancements in nanoelectronics, sensors, and biomedical devices.
Understanding and manipulating impurities in materials are central to advancing technology, enabling tailored materials with specific properties vital across industries. The deliberate incorporation and control of impurities empower innovation and drive the development of materials critical for the advancement of society and various technological domains.
Conclusion
Impurities significantly influence material properties across industries, shaping the functionality of materials in electronics, metallurgy, medicine, and beyond. Their deliberate control and incorporation enable tailored materials with enhanced properties, driving innovation and technological advancements essential for modern society’s progress and diverse applications.
Understanding impurities’ role and harnessing their potential pave the way for continuous innovation and the development of materials crucial for evolving technologies and industries.