Geometries of Molecular Geometry and Electron Geometry are the fundamental ideas within the realm of chemistry. They offer insights into the atomic arrangement in three dimensions in a molecule, as well as their distribution around an atom. Geometries that are governed by the Valence Shell Electron Pair Repulsion (VSEPR) theory are crucial in determining the molecule’s chemical and physical properties.
While electron geometry concentrates on bonding and single electron pairs molecular geometry only considers the arrangement of atoms frequently resulting in different shapes. Understanding the difference between these two concepts is vital to predicting molecular behavior interaction, reactivity, and reactivity.
Basics of VSEPR Theory
-
- Introduction
- Defined: VSEPR stands for Valence Shell Electron Pair Repulsion Theory. It’s a mathematical model that helps predict the structure of molecules, based on the attraction between electron pairs inside the Valence Shell of the central atom.
- Core Principles
- Electron Pair Repulsion: Electron pairs either as bonds (bonding pairs) or on their own (lone pairs) are attracted by each other. They adapt their positions to remain as separated as possible, which reduces Repulsion and thereby stabilizes the molecules.
- Electron Pair arrangement: The spatial arrangement of electron pairs around the center of the atom determines the electron geometry of the molecule.
- Lone Pairs vs. Bonding Pairs: Single pairs are more prone to repel as bonding couples. This implies it is possible that the presence of isolated pairs could alter the molecular shape from its electron-based geometrical.
- Common Electron Pair Arrangements & Resulting Molecular Geometries
- Linear: Two electron pairs Some examples include BeCl2.
- Trigonal Planar: Three electron pairs Some examples include BF3. When only one pair occurs, the molecular structure changes and the shape is altered or angular like in SO2.
- Tetrahedral: Four electron pair Examples include CH4. When there is just one pair, the form changes to trigonal pyramidal (NH3) when it is joined by two pairs of lone pairs, it turns bent or angulated (H2O).
- Trigonal Bipyramidal: Five electrons Examples include PCl5. The variations caused by the lone pair can result in forms like T-shaped, see-saw, and linear.
- Octahedrals: 6 electron pairs Examples include the SF6. When lone pairs are present, shapes could be square pyramidal or square planar.
- Factors Affecting Repulsion Strength
- Lone pair-lone Pair (LP-LP) Repulsion: The most powerful type of repulsion is due to the fact that the lone pair occupies more space.
- Lone Couple-Bonding Pair (LP-BP) Repulsion: The strength is moderate.
- Bonding Pair-Bonding Pair (BP-BP) Repulsion: This is the least effective form of repulsion.
- Limitations of VSEPR Theory
- VSEPR is a simplified method and does not always reflect all molecular shapes, but it is particularly true for bigger molecules or those that have orbitals d and f.
- VSEPR does not provide an explanation of the source of the forms but rather provides them with a description.
- VSEPR theory is an effective instrument for predicting and understanding molecular structures. By analyzing the quantity and nature of electron pairs, VSEPR theory offers an understanding of molecular geometry. It can play a significant part in determining a molecule’s properties Reactivity, properties, and interactions.
What is Electron Geometry?
Electron geometry is the arrangement of spatial space for the electron pairs (both bonds and lone pairs) around the central atom within an ion or molecule. It’s based primarily on the notion the electrons, because of their charge negative, will repel each other, and consequently be arranged in a way that reduces this repelling. This idea is the basis of the theory of VSEPR (Valence Shell Electron Pair Repulsion) theory.
Electron Pairs Involved:
- Electron geometry includes both bonding electron pairs (those that are shared by two atoms of bonds) and single pairs (those that are not involved in bonding but are only localized to one atom).
Common Electron Geometries:
- Linear: Two pairs of electrons (could comprise two bonding pairs or a bonding pair, and one pair that is lone). Example: BeCl2.
- Trigonal Planar: three electron pair (could comprise three bonds, or a combination of bonding and singular pairs). Example: BF3.
- Tetrahedral: Four pairs of electrons. Example: CH4.
- Trigonal Bipyramidal: Five pairs of electrons. Example: PCl5.
- Octahedral: 6 electron-electron pairs. Example: SF6.
Determinants of Electron Geometry:
- The main determinator is the total number of electron pairs that surround the atom’s central region. This includes both bonds that bond the pairs as well as the single pair.
- The geometry or shape results from these pairs of electrons arranging themselves as far from one of their counterparts as they can, thus minimizing the chance of repulsion.
Different from Molecular Geometry:
- While electron geometry is focused exclusively on the configuration of every electron pair molecular geometry is the arrangement of just the atoms of the molecule. Since lone pairs aren’t evident in the molecular form however they can influence the geometries and shape, molecular geometry may differ from electron geometry.
Significance of Electron Geometry:
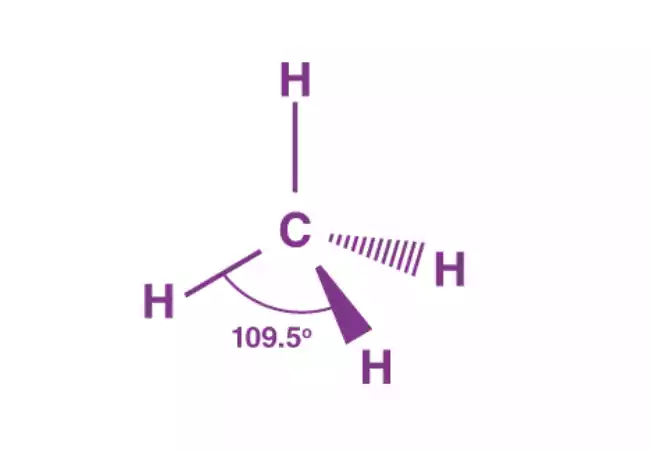
Knowing the electron’s geometry can be essential in determining the molecular shape of molecules. It also provides insight into the distribution of electrons around the central atom that can affect the properties of polarity, reactivity, and a variety of other molecular characteristics.
What is Molecular Geometry?
The term “molecular geometry,” often known as molecular form describes the three-dimensional arrangement of atoms inside molecules. It provides a concrete representation of the spatial relations between these atoms. It can provide crucial insights into the properties of a molecule, its behavior, and its reactions.
Key Aspects of Molecular Geometry:
Determined by Electron Pairs:
- While molecular geometry is focused on the location of atoms, the arrangement of atoms is determined by the electron pairs (both bonding and lone pair) in the atom’s center. The pairs are prone to resent each other by repositioning themselves in a way to reduces this repelling.
Common Molecular Geometries:
- Linear: Atoms can be arranged into straight lines. Example: CO2.
- Bent, or Angular: Atoms create an angle, which is typically observed when there are single pairs of atoms in the center. Example: H2O.
- Trigonal Planar: Atoms are located within the same plane and form the triangular form. Example: BF3.
- Triangle Pyramidal: An elongated pyramid with an equilateral triangular base. Example: NH3.
- Tetrahedral: Axons are formed into a shape that resembles a pyramid, but with a triangular base. Example: CH4.
- Trigonal Bipyramidal T-shaped, See-saw: Variations that are observed where there exist five electronic densities surrounding the core electron. For example: PCl5 for trigonomic bipyramidal.
- Octahedral Square Planar, Square Pyramidal: Shapes derived from six electron density regions in the central electron. For example: SF6 is for octahedral.
Influence of Lone Pairs:
- The electrons are the only ones in the space around them and are able to repel bonding pair, however, they’re not part of the structure of molecular molecules “visible structure.” This means that they may alter the shape of molecular geometry. For instance, both H2O as well as CH4 have tetrahedral electrons however H2O is a bent molecular shape because of the presence of two pairs of lone pairs that are located that are located on oxygen.
Different from Electron Geometry
- The electron geometry is a consideration of the electron pair in all its forms (bonding and single pairs) molecular geometry examines the actual positions that the electrons are in. Therefore, there are lone pair structures that could create a different molecular structure from electron geometry.
Significance of Molecular Geometry:
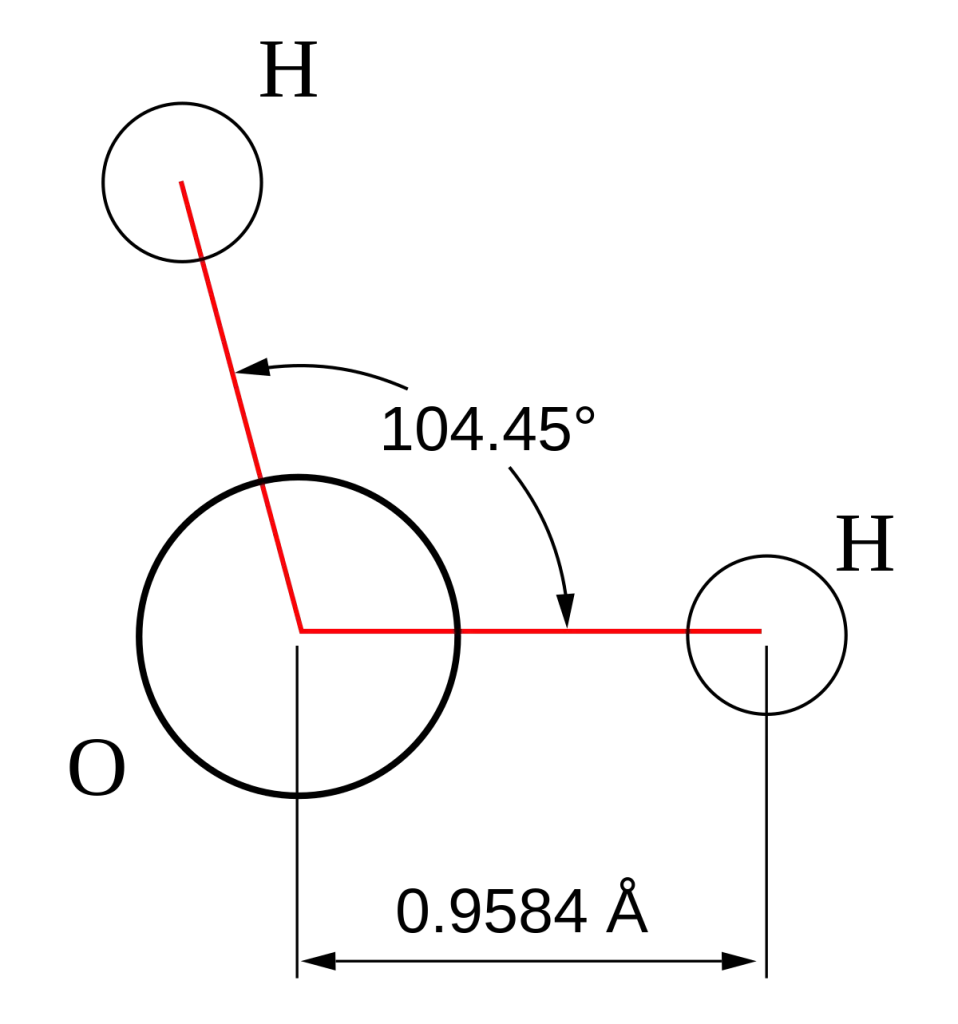
The shape of molecules is a key factor in controlling many of their characteristics like polarity, the phase of matter, reactivity, and color, as well as magnetism as well as biological activities. For example, understanding molecular geometry is essential in the field of drug design and drug development, where there is a “fit” between a drug molecule and the protein that it is targeting is like an individual key being fitted to locks.
Molecular Geometry and Electron Geometry in the comparative chart
Comparative Chart: Molecular Geometry vs. Electron Geometry
Aspect | Molecular Geometry | Electron Geometry |
Definition | Describes the spatial arrangement of atoms in a molecule. | Describes the spatial arrangement of all electron pairs (bonding and lone pairs) around a central atom. |
Based on | Positioning of atoms only. | Positioning of both bonding and lone electron pairs. |
Factors Influencing | Number and location of bonding atoms. | Number and location of both bonding pairs and lone pairs. |
Affected by Lone Pairs? | Yes, they can change the shape from the electron geometry but are not seen in the shape itself. | Yes, they occupy space and determine the geometry but aren’t part of the shape’s naming. |
Examples | Bent, Linear, Tetrahedral, Trigonal Pyramidal, Trigonal Planar, etc. | Linear, Trigonal Planar, Tetrahedral, and Octahedral arrangements exist for any given environment. |
Relevance to Polarity | The shape can determine if a molecule is polar or non-polar, depending on atom distribution. | Gives insight but may not directly determine polarity, as the shape can differ due to lone pairs. |
Importance | Critical for understanding intermolecular forces, reactivity, and molecular properties. | Fundamental for predicting molecular geometry and understanding electronic distribution. |
This chart provides a concise comparison of the two concepts, highlighting their differences and similarities.
Importance in understanding molecular shape and reactivity
-
- Molecular Polarity:
-
- The shape of the molecule plays an important role in the determination of its degree of polarity. Polar molecules have a dispersed electron distribution, resulting in a molecule that has both a positive and negative side (or the poles).
- For example, the bent form that water has (H2O) results in the formation of a negative and positive pole, which makes it a bipolar molecule. This polarity is the reason for the high boiling point of water surface tension, as well as the capacity to dissolve many different substances.
- Intermolecular Forces:
-
- The shape of molecules affects the nature and force of intermolecular forces (e.g. hydrogen bonding van der Waals forces and dipole-dipole interaction).
- Forces that are stronger between the molecules lead to higher melting and boiling temperatures and could affect the solubility.
- Chemical Reactivity:
-
- The reactivity of a molecule usually depends on the orientation of its sites for reactivity. Molecules that have specific shapes may reveal or block certain sites, altering the way they react with other molecules.
- Biology is one of the most prominent examples where the shape of the molecular (substrate) must match the structure of its enzyme to allow a reaction to take place.
- Stereochemistry and Isomerism:
-
- Understanding the shape of molecular molecules is crucial to understanding stereochemistry, which is the study of spatial arrangements of atoms inside molecules.
- Isomers may share identical molecular formulas however different spatial or structural arrangements, which can result in distinct chemical and physical properties.
- Molecular Function in Biological Systems:
-
- The effectiveness of a number of biomolecules, such as enzymes, receptors, and antibodies is mostly determined by their form. A “lock and key” model of enzyme activity is an excellent example of how it is the form of an enzyme (lock) must correspond to that of its substrate (key).
- Drug Design and Medicinal Chemistry:
-
- Knowing molecular structure in the pharmaceutical sector is vital to developing drugs that work with specific biological molecules, maximizing therapeutic effects, and minimizing adverse side effects.
- Catalysis:
-
- Catalysts function by providing an environment that allows reactants to join in a favorable position to begin a reaction. The form and shape of a catalyst could affect the speed and selectivity of the reaction.
- Electronic Configuration and Molecular Orbital Theory:
-
- The shape of a molecular molecule can offer clues into the electronic structure of a molecule.
- Molecular Orbital (MO) theory is the theory that describes the development of molecular orbitals due to the overlapping of orbitals in atomic form and is intimately connected to the shape and chemical reactivity of molecules.
Understanding the shape of a molecule is crucial in determining how a molecule will behave both physically and chemically. It serves as a foundation for the many phenomena that occur in nature. It also plays a role in the advancement of fields such as biochemistry, medicine as well and materials sciences.
Applications and Implications of Molecular Geometry and Electron Geometry
Understanding electron and molecular geometries can have profound impacts in a variety of areas of science and technology.
Here’s a look at their importance:
Chemistry & Reactivity
- The ability to predict reactivity: Reactivity in molecules usually depends on their shape. For instance, molecules that have particular orientations could permit (or hinder) certain kinds of reactions.
- Stereochemistry: The role of molecular geometry is crucial in understanding isomerism, specifically stereoisomerism. Isomers such as trans and E and Z differ by spatial arrangement.
Biology & Medicine
- Enzyme Function: This “lock and key” model of enzymes suggests that the enzymes (locks) are particular to specific substrates (keys) in relation to molecular structure.
- Drug Design: In pharmacology understanding the molecular anatomy of active sites as well as potential drugs is crucial. The drugs must “fit” their target sites within the body. This is usually it’s a matter of form and orientation.
- Cell receptors: Cell receptors’ function is defined by their shape, which permits them to communicate with certain molecules.
Material Science
- Polymers: Properties of polymers including their flexibility and strength, can be affected by the shape of monomer structures, as well as how they are laid out in space.
- Crystallography: Crystallography is the study of structures in crystals and how they function. It is grounded in the understanding of how spatial arrangements are made by molecules and atoms.
Environmental Science
- Greenhouse Gases: The shape of molecules such as CH4, CO2, and water vapor affects their capacity to absorb and emit infrared radiation which is a factor in climate change.
- Pollutant Behavior: The behavior and reactivity of pollutants regardless of whether they are in air, water, or in soil, are determined by their molecular forms.
Physical Properties & Phenomena
- Polarity and Intermolecular Forces: Molecular geometry determines the degree to which a molecule is polar and can affect the type and force of intermolecular forces that in turn affect aspects like melting points or boiling points, viscosity, as well as solubility.
- Optic activity: Compounds that can move the plane of polarized light, referred to as chiral compounds, owe their capability to their unique spatial arrangement of the atoms.
Electronics & Nanotechnology
- Semiconductors: The arrangement and geometry of molecules and atoms in semiconductors affect their conductivity properties.
- Molecular Machines: Nanotechnology is the development of molecular devices that require an understanding of the electron and molecular geometries in order to guarantee the functionality you want.
Astrochemistry & Cosmology
- Exoplanet Atmospheres: Understanding the molecular and electron structure of molecules that could be found in the atmospheres of exoplanets can give insight into their properties and the potential for the development of life.
- Interstellar Medium: The behavior and interactions of molecules across Space can be predicted by their shape and distribution of electrons.
The arrangement of electrons and atom pairs in molecules plays an essential part in understanding myriad processes, properties, and phenomena in a myriad of fields of study. Understanding the geometries of molecules is an essential step to predicting and understanding molecular interactions and behavior.
Real-world Examples
Real-world examples show the significance of electron and molecular geometries in the real world.
Here are some examples in diverse fields:
Pharmaceuticals and Medicine:
- Thalidomide Tragedy: Thalidomide is a medication that is available in two forms of stereoisomeric because of different molecular geometries. The first type (R-enantiomer) was conceived as a treatment for morning sickness during pregnancy, however, the second form (S-enantiomer) resulted in serious birth defects. The inability to differentiate the two types at first, or understand their differing biological effects had devastating consequences.
Chemical Sensors:
- Carbon Monoxide Detectors: The geometrical structure of CO allows it to bond tightly to hemoglobin, which blocks oxygen transportation in blood. CO detectors, crucial in the home, detect and react when they detect the existence of this chemical, thereby preventing CO poisoning.
Food Industry:
- Artificial sweeteners: The molecular shape of molecules such as aspartame is like natural sugars, which allows them to be incorporated into those same receptors for taste that are located on our tongues, causing them to taste sweet. Because of different molecular shapes, our bodies process these sweeteners in different ways.
Environmental Concerns:
- CFCs, Ozone Depletion: The shape of the chlorofluorocarbons (CFCs) allows them to remain stable and not reactive within the lower atmosphere, but damaging to the ozone stratosphere. This understanding has led to international agreements that allowed for the gradual elimination of CFC production.
Perfume Industry:
- Musk Molecules: The shape and form of molecules determine their smell. Musk molecules, whether they are natural or synthetic, possess specific shapes that connect to the olfactory receptors. They give them a distinctive scent.
Agriculture:
- Herbicides and Pesticides: The molecular form of these chemicals determines their efficacy. Certain chemicals are specially designed to work with the biochemical pathway of the pests and herbicides, which inhibit their growth and development without adversely impacting crops.
Material Science:
- Liquid Crystals: They are used for LCD (Liquid Crystal Display) screens, these substances contain specific molecular geometries that allow the material to be aligned in particular patterns when an electrical field is applied to them, which controls light flow and creates images that screen.
Art Restoration and Forensics:
- Pigment Analysis: The molecular form of pigments may affect their color and the way they react with light. Through understanding the molecular structure of the various pigments, artists can identify the materials originally used in art as well and forensic scientists can identify the materials that are discovered at crime scenes.
The electron and molecular geometries of compounds are not only academic concepts, they are essential in understanding how to design, analyze, and respond to the multitude of real-world scenarios and problems.
Conclusion
The geometries of electron geometry and molecule geometry are the foundational elements in understanding the behavior and interactions of molecules within the world that surrounds us. From the drugs we consume the screens we use to watch and even the air we breathe the arrangement of electrons and atom pairs determines their characteristics, reactivities, and functions.
With these ideas, Scientists and professionals from different fields can invent solve problems, discover and harness the potential of molecules to improve our daily lives as well as address global issues.