If You Are in the Field of Biochemistry, Molecular Biology, or Genetics, You May Have Come Across the Terms Radioactive and Nonradioactive Probes. These Probes Are Used Extensively in the Detection and Analysis of DNA, RNA, and proteins.
But What Exactly Are These Probes, and What Are the Differences Between Them? in This Article, We Will Explore the Difference Between Radioactive and Nonradioactive Probes, Their Uses, Advantages, and disadvantages.
Introduction of Radioactive and Nonradioactive Probes
Probes Are Molecules That Are Used to Detect Specific Nucleic Acid Sequences or Proteins. These Molecules Bind to the Target Molecule, Allowing Researchers to Locate and Identify It. Radioactive Probes and Nonradioactive Probes Are Two Types of Probes That Are Commonly Used in Molecular biology.
Radioactive Probes Are Labeled With a Radioactive Isotope, Which Emits Radiation That Can Be Detected by Autoradiography. Nonradioactive Probes, on the Other Hand, Are Labeled With a Nonradioactive Molecule, Such as a Fluorescent Dye or Biotin, Which Can Be Detected by Different techniques.
Radioactive Probes
Radioactive Probes Are a Type of Molecular Tool Used in Scientific Research, Diagnostics, and Various Fields to Detect, Track, and Analyze Specific Molecules or Biological Processes. These Probes Incorporate Radioactive Isotopes, Which Emit Radiation That Can Be Detected and Measured.
Here Are Some Key Aspects of Radioactive probes:
1. Definition And Characteristics:
• Radioactive Probes Are Composed of a Molecule (e.g., DNA, RNA, Antibody) Labeled With a Radioactive isotope.
• The Radioactive Isotope Undergoes Radioactive Decay, Emitting Radiation in the Form of Alpha Particles, Beta Particles, or Gamma rays.
• Common Radioactive Isotopes Used in Probes Include ^32P, ^35S, ^3H, and ^125I.
2. Applications in Various Fields:
• Medicine (Nuclear Medicine, Diagnostic Imaging): Radioactive Probes Are Used in Techniques Such as Positron Emission Tomography (PET), Single-Photon Emission Computed Tomography (SPECT), and radioimmunoassays.
• Molecular Biology: They Play a Crucial Role in DNA Sequencing, Gene Expression Analysis, and Studying Protein-Protein interactions.
• Environmental Monitoring: Radioactive Probes Help Track and Analyze Pollutants, Study Radioactive Contamination, and Understand Geological processes.
3. Advantages of Radioactive Probes:
• High Sensitivity and Resolution: Radioactive Decay Emits Highly Detectable Radiation, Enabling Precise Measurements and Trace-Level detection.
• Wide Range of Isotopes Available: Different Isotopes With Varying Decay Properties Allow for Diverse Applications and Experimental flexibility.
4. Limitations and Concerns:
• Radioactivity Hazards: Working With Radioactive Materials Carries Risks to Researchers and Requires Adherence to Strict Safety protocols.
• Limited Half-Life: Many Radioactive Isotopes Have Short Half-Lives, Limiting the Duration of Their Use and Necessitating Frequent replenishment.
• Regulatory Restrictions: The Use, Handling, and Disposal of Radioactive Materials Are Regulated by Governmental Bodies to Ensure Safety and Prevent Environmental contamination.
Despite Their Widespread Use, the Potential Risks Associated With Radioactive Probes Have Led to the Development and Adoption of Alternative Nonradioactive Probes, Such as Fluorescent and Enzymatic probes.
These Alternatives Offer Improved Safety Profiles, Longer Shelf Life, and Expanded Imaging Options. Nonetheless, Radioactive Probes Remain Valuable Tools in Specific Applications Where Their Unique Properties and High Sensitivity Are Required.

Advantages of Radioactive probes
Radioactive Probes Offer Several Advantages in Scientific Research and Diagnostics Due to Their Unique Properties.
Here Are Some Key Advantages of Radioactive probes:
1. High Sensitivity: Radioactive Probes Emit Radiation During Radioactive Decay, Which Can Be Easily Detected and Quantified. This High Sensitivity Allows for the Detection of Low Levels of Target Molecules or Biological Processes, Even in Complex samples.
2. Quantitative Analysis: Radioactive Probes Can Provide Quantitative Measurements Due to the Ability to Directly Count Emitted Radiation. This Enables Precise Determination of Target Concentrations and Allows for Accurate Comparisons Between Different samples.
3. Versatility: Radioactive Probes Can Be Used in a Wide Range of Applications and Experimental Techniques. They Are Utilized in Fields Such as Molecular Biology, Medicine, Environmental Monitoring, and More. Different Radioactive Isotopes With Varying Decay Characteristics Provide Versatility in Experimental Design and customization.
4. Signal Stability: The Radiation Emitted by Radioactive Probes Is Stable Over Time, Making It Possible to Analyze Samples Even After a Considerable Duration. This Stability Allows for Longer Experimental Durations and the Ability to Revisit and Reanalyze Samples if needed.
5. Subcellular Localization: Radioactive Probes Can Be Used to Track and Study Cellular Processes at a Subcellular Level. for Example, by Labeling Specific Molecules With Radioactive Isotopes, Researchers Can Observe Their Movement Within Cells or Trace Their Interaction With Cellular components.
6. Imaging Capabilities: In Medical Imaging, Radioactive Probes Are Utilized in Techniques Such as PET and SPECT Scans. These Imaging Methods Provide Detailed Information About Physiological and Biochemical Processes in the Body, Aiding in Disease Diagnosis, Monitoring Treatment Response, and Research studies.
7. Established Methods and Infrastructure: The Use of Radioactive Probes Has a Long History and Well-Established Protocols, Making It Easier to Implement Them in Research and Clinical Settings. Additionally, There Is Existing Infrastructure, Including Specialized Equipment and Trained Personnel, to Handle Radioactive Materials and Conduct Experiments safely.
It Is Important to Note That While Radioactive Probes Offer Significant Advantages, They Also Come With Certain Limitations and Safety considerations. These Include Potential Hazards Associated With Radioactivity, Limited Half-Life of Some Isotopes, and Regulatory restrictions. The Choice of Probe Should Be Based on the Specific Requirements of the Experiment or Application, Considering Both the Advantages and Potential Drawbacks.
Limitations and concerns
While Radioactive Probes Have Advantages, They Also Come With Certain Limitations and Concerns. It Is Important to Be Aware of These Factors When Considering the Use of Radioactive Probes in Scientific Research and Diagnostics.
Here Are Some Key Limitations and Concerns Associated With Radioactive probes:
1. Radioactivity Hazards: Working With Radioactive Materials Poses Potential Health Hazards to Researchers and Requires Adherence to Strict Safety Protocols. Exposure to Radiation Can Have Detrimental Effects on Human Health, Including Increased Risk of Cancer and Other Radiation-Related Illnesses. Proper Handling, Storage, and Disposal Procedures Must Be Followed to Minimize risks.
2. Limited Half-Life: Many Radioactive Isotopes Used in Probes Have Short Half-Lives, Meaning They Decay Relatively Quickly. This Limits the Duration of Their Usefulness and Necessitates Frequent Replenishment of Probes for Continued Experiments. Researchers Must Plan Experiments Carefully to Ensure They Can Be Completed Within the Available Half-Life of the Radioactive isotope.
3. Regulatory Restrictions: The Use, Handling, and Disposal of Radioactive Materials Are Regulated by Governmental Bodies to Ensure Safety and Prevent Environmental Contamination. Researchers Must Comply With These Regulations and Obtain the Necessary Permits and Licenses to Work With Radioactive Probes. This Can Add an Administrative Burden and May Require Specialized Training and facilities.
4. Instrumentation Requirements: Detection and Measurement of the Radiation Emitted by Radioactive Probes Typically Require Specialized Instrumentation, Such as Scintillation Counters or Gamma Counters. These Instruments Can Be Expensive, and Their Maintenance and Calibration Need to Be Carried Out Regularly to Ensure Accurate results.
5. Cost Considerations: Radioactive Probes Can Be Costly Due to the Need for Handling and Disposal of Radioactive Materials, Regulatory Compliance, and Specialized Instrumentation. the Costs Associated With Purchasing and Maintaining Radioactive Isotopes Can Be Significant, Especially for Long-Term Experiments or Large-Scale studies.
6. Environmental Impact: Radioactive Materials Can Pose Risks to the Environment if Not Handled and Disposed of Properly. It Is Crucial to Follow Appropriate Protocols for the Safe Storage, Transportation, and Disposal of Radioactive Waste to Prevent Contamination of Soil, Water, and ecosystems.
7. Public Perception and Acceptance: The Use of Radioactive Probes Can Raise Concerns Among the Public and May Face Public Scrutiny Due to the Association of Radioactivity With Potential Harm. Researchers and Practitioners Must Communicate Effectively About the Safety Measures in Place to Address These Concerns and Ensure Public Trust in the Responsible Use of Radioactive probes.
Considering These Limitations and Concerns, It Is Important for Researchers to Carefully Assess the Necessity and Suitability of Radioactive Probes for Their Specific Experiments or applications. Alternative Nonradioactive Probes, Such as Fluorescent or Enzymatic Probes, May Offer Safer and More Practical Options in Certain Cases.
Nonradioactive Probes
Nonradioactive Probes, Also Known as Nonisotopic Probes, Are Molecular Tools Used in Scientific Research and Diagnostics to Detect, Track, and Analyze Specific Molecules or Biological Processes Without the Use of Radioactive Isotopes. These Probes Offer Several Advantages, Including Improved Safety, Longer Shelf Life, and Expanded Imaging Options.
Here Are Some Key Aspects of Nonradioactive probes:
1. Definition And Characteristics:
• Nonradioactive Probes Are Typically Labeled With Nonradioactive Markers, Such as Fluorescent Dyes, Enzymes, or Chemical tags.
• These Markers Emit or Produce Detectable Signals That Can Be Visualized or Measured Using Appropriate techniques.
2. Types of Nonradioactive Probes:
• Fluorescent Probes: These Probes Utilize Fluorescent Dyes or Fluorophores That Emit Light When Excited by a Specific Wavelength of Light. They Are Widely Used in Microscopy, Flow Cytometry, and Fluorescent-Based assays.
• Enzymatic Probes: These Probes Rely on Enzymes That Catalyze Specific Reactions, Resulting in the Generation of a Detectable Signal. Examples Include Enzyme-Linked Immunosorbent Assays (ELISA) and Enzyme-Based Detection systems.
• Chemical Probes: These Probes Utilize Chemical Tags or Markers That Undergo Specific Chemical Reactions, Enabling Their Detection or Visualization. Examples Include Biotin-Streptavidin Labeling Systems and Hybridization probes.
3. Applications in Various Fields:
• Fluorescent Microscopy: Nonradioactive Fluorescent Probes Are Extensively Used to Visualize and Study Cellular Structures, Protein Localization, and Dynamic Processes Within cells.
• Protein and Cell Labeling: Nonradioactive Probes Are Employed to Label and Track Proteins or Cells in Research Studies, Drug Discovery, and Diagnostic applications.
• DNA and RNA Detection: Nonradioactive Probes Enable the Detection and Quantification of DNA and RNA Sequences, Aiding in Gene Expression Analysis, Genotyping, and Nucleic Acid-Based diagnostics.
4. Advantages of Nonradioactive Probes:
• Improved Safety: Nonradioactive Probes Eliminate the Potential Health Risks Associated With Working With Radioactive Materials, Making Them Safer for Researchers and Minimizing Environmental concerns.
• Longer Shelf Life: Nonradioactive Probes Generally Have Longer Stability, Allowing for Extended Storage and Use Without Significant Degradation or decay.
• Versatility and Compatibility: Nonradioactive Probes Can Be Used in Various Experimental Techniques and Are Compatible With a Wide Range of Detection Instruments and Imaging systems.
• Multiple Labeling Options: Nonradioactive Probes Offer a Diverse Selection of Markers, Allowing for Multiplexing—Simultaneous Detection of Multiple Targets Using Different markers.
5. Limitations and Challenges:
• Lower Sensitivity Compared to Radioactive Probes: Nonradioactive Probes Typically Have Lower Sensitivity and May Require Amplification Techniques to Enhance Signal Detection, Especially When Working With Low-Abundance targets.
• Photobleaching and Background Noise: Fluorescent Probes Can Suffer From Photobleaching, Leading to a Reduction in Signal Intensity Over Time. Additionally, Nonradioactive Probes May Produce Background Noise, Affecting the Signal-to-Noise Ratio in Certain assays.
Nonradioactive Probes Have Gained Popularity and Wide Adoption in Scientific Research and Diagnostics Due to Their Safety, Versatility, and Compatibility With Modern Imaging and Detection technologies. They Offer Viable Alternatives to Radioactive Probes in Many Applications, Providing Researchers With Effective and Reliable Tools for Their Experiments and Diagnostic Assays.
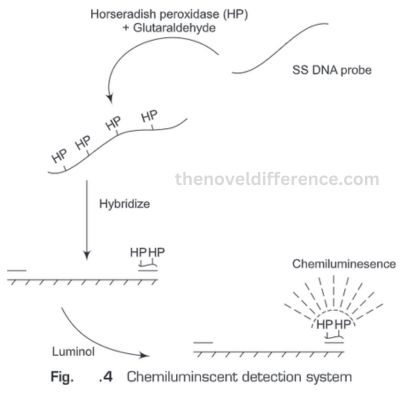
Advantages of Nonradioactive probes
Nonradioactive Probes, Also Known as Nonisotopic Probes, Offer Several Advantages in Scientific Research and Diagnostics.
Here Are Some Key Advantages of Nonradioactive probes:
1. Improved Safety: Nonradioactive Probes Eliminate the Potential Health Risks Associated With Working With Radioactive Materials. This Makes Them Safer for Researchers, Laboratory Personnel, and Patients Undergoing Diagnostic Procedures. the Use of Nonradioactive Probes Reduces the Need for Stringent Radiation Safety Protocols and Minimizes the Potential for Environmental contamination.
2. Longer Shelf Life: Nonradioactive Probes Generally Have Longer Stability Compared to Radioactive Probes. They Can Be Stored for Longer Periods Without Significant Degradation or Decay, Allowing Researchers and Diagnostic Laboratories Greater Flexibility in Experimental Planning and Reducing the Need for Frequent Replenishment of probes.
3. Versatility and Compatibility: Nonradioactive Probes Are Compatible With a Wide Range of Experimental Techniques, Instruments, and Detection Systems. They Can Be Used in Various Fields of Study, Including Molecular Biology, Cell Biology, Immunology, and Diagnostics. Researchers Can Choose From a Diverse Selection of Nonradioactive Markers, Allowing for Flexibility in Experimental Design and customization.
4. Multiple Labeling Options: Nonradioactive Probes Offer a Variety of Labeling Options, Such as Fluorescent Dyes, Enzymes, Chemical Tags, or Nanoparticles. This Enables Researchers to Perform Multiplexing Experiments, Simultaneously Detecting and Analyzing Multiple Targets or Markers in a Single Sample. Multiplexing Expands the Information Obtained From an Experiment and Enhances efficiency.
5. Signal Stability and Sensitivity: Nonradioactive Probes, Particularly Fluorescent Probes, Can Provide Stable and Long-Lasting Signals. Advances in Fluorescent Dye Technology Have Improved Their Photostability, Reducing Photobleaching and Ensuring Signal Integrity Over Extended Imaging or Detection Periods. Additionally, Signal Amplification Techniques Can Be Employed to Enhance Sensitivity and Improve the Detection Limits of Nonradioactive probes.
6. Real-Time Imaging and Visualization: Nonradioactive Probes, Such as Fluorescent Probes, Enable Real-Time Imaging and Visualization of Cellular Structures, Processes, and Interactions. This Allows Researchers to Observe Dynamic Changes Within Living Cells, Track Molecular Events, and Study Cellular Responses in Real-Time. Nonradioactive Probes Facilitate High-Resolution Imaging Techniques, Such as Fluorescence Microscopy, Confocal Microscopy, and Live-Cell imaging.
7. Ease of Use and Cost-Effectiveness: Nonradioactive Probes Are Generally Easier to Handle, as They Do Not Require Specialized Radiation Safety Protocols or Dedicated Facilities. They Also Eliminate the Need for Expensive Radiation Detection and Measurement Instruments Associated With Radioactive Probes. This Ease of Use and Reduced Infrastructure Requirements Make Nonradioactive Probes More Accessible and Cost-Effective for Many Research Laboratories and Diagnostic settings.
Considering These Advantages, Nonradioactive Probes Have Become Widely Adopted in Various Scientific Fields and Diagnostic applications. They Provide Reliable and Safer Alternatives to Radioactive Probes, Offering Researchers and Healthcare Professionals Effective Tools for Their Experiments and Diagnostic Assays.
Limitations and challenges
While Nonradioactive Probes Offer Several Advantages, They Also Have Some Limitations and Challenges. It’s Important to Consider These Factors When Utilizing Nonradioactive Probes in Scientific Research and Diagnostics.
Here Are Some Key Limitations and Challenges Associated With Nonradioactive probes:
1. Lower Sensitivity: Nonradioactive Probes Generally Exhibit Lower Sensitivity Compared to Radioactive Probes. This Can Pose Challenges When Detecting Low-Abundance Targets or When Working With Samples Containing Low Concentrations of the Molecule of Interest. Signal Amplification Techniques, Such as Enzyme Amplification or Signal Amplification Systems, May Be Required to Enhance sensitivity.
2. Background Noise: Nonradioactive Probes, Especially Fluorescent Probes, May Generate Background Noise or Autofluorescence in Biological Samples. This Can Reduce the Signal-to-Noise Ratio and Impact the Accuracy and Specificity of Detection. Careful Optimization of Experimental Conditions, Including Proper Selection of Filters, Controls, and Background Reduction Strategies, Is Necessary to Minimize Background noise.
3. Photobleaching: Fluorescent Probes Are Susceptible to Photobleaching, Which Is the Irreversible Loss of Fluorescence Signal Due to Prolonged Exposure to Excitation Light. This Can Limit the Imaging or Detection Duration and May Require Strategies Such as Using Photostable Fluorophores, Optimizing Imaging Parameters, and Implementing Image Acquisition and Analysis Techniques to Mitigate Photobleaching effects.
4. Instrumentation Compatibility: Nonradioactive Probes Often Require Specialized Instrumentation for Detection and Analysis, Such as Fluorescence Microscopes, Plate Readers, or Imaging Systems. These Instruments Can Be Costly, and Access to Them May Be Limited in Certain Research or Clinical Settings. Researchers Need to Ensure the Availability and Compatibility of the Required Equipment for Their Specific Nonradioactive Probe-Based assays.
5. Multiplexing Limitations: While Nonradioactive Probes Offer the Possibility of Multiplexing—Simultaneous Detection of Multiple Targets—They May Have Limitations in Terms of the Number of Targets That Can Be Effectively Detected and Resolved. Overlapping Emission Spectra or Spectral Bleed-Through Between Fluorophores Can Hinder Accurate Multiplexing, Requiring Careful Selection and Optimization of Fluorophores and Detection methods.
6. Validation and Standardization: Nonradioactive Probe-Based Assays Often Require Rigorous Validation and Standardization to Ensure Reliability, Accuracy, and Comparability of Results. This Includes Optimizing Assay Conditions, Establishing Appropriate Controls, and Validating the Performance of the Assay Across Different Samples and Experimental setups.
7. Availability of Specific Probes: Depending on the Specific Target or Application, Suitable Nonradioactive Probes May Not Always Be Readily Available. Researchers May Need to Develop or Customize Their Own Probes, Which Requires Expertise in Probe Design, Synthesis, and characterization.
Despite These Limitations and Challenges, Nonradioactive Probes Have Proven to Be Valuable Tools in Many Research and Diagnostic applications. With Careful Experimental Design, Optimization, and Proper Controls, These Probes Can Provide Reliable and Meaningful Results While Offering Improved Safety and Versatility Compared to Radioactive Probes.
Difference Between Radioactive and Nonradioactive Probes
The Difference Between Radioactive and Nonradioactive Probes Lies Primarily in the Type of Marker or Label Used to Detect and Track Specific Molecules or Biological Processes.
Here Are the Key Differences Between Radioactive and Nonradioactive probes:
Radioactive Probes:
1. Marker: Radioactive Probes Use Radioactive Isotopes, Such as ^32P, ^35S, or ^125I, as the Labeling Component. These Isotopes Emit Radiation as They Undergo Radioactive decay.
2. Detection Method: The Emitted Radiation From Radioactive Probes Is Detected Using Specialized Equipment, Such as Scintillation Counters or Autoradiography. the Radiation Is Quantified to Measure the Presence or Concentration of the Target molecule.
3. Sensitivity: Radioactive Probes Are Highly Sensitive, Allowing for the Detection of Low Levels of Target Molecules Due to the Ability to Directly Count Emitted Radiation. This Makes Them Useful for Applications Requiring High Sensitivity, Such as Tracking Trace Amounts of Specific Molecules in Biological samples.
4. Quantification: Radioactive Probes Provide Quantitative Measurements as the Emitted Radiation Can Be Directly Counted and Correlated With the Amount of Target Molecule Present. This Enables Accurate Determination of Concentrations and Comparisons Between Different samples.
5. Half-Life: Radioactive Isotopes Have Specific Half-Lives, Which Determine the Duration of Their Usefulness. Some Isotopes Have Short Half-Lives, Requiring Frequent Replenishment of Probes, While Others Have Longer Half-Lives for More Extended Experimental durations.
Nonradioactive Probes:
1. Marker: Nonradioactive Probes Use Nonradioactive Markers, Such as Fluorescent Dyes, Enzymes, Chemical Tags, or Nanoparticles. These Markers Produce Detectable Signals (e.g., Fluorescence or Enzymatic Reactions) Without Emitting radiation.
2. Detection Method: Nonradioactive Probes Are Detected Using Techniques Specific to the Marker Used. for Example, Fluorescence-Based Probes Are Visualized Using Fluorescence Microscopy or Measured Using Fluorescence Plate readers.
3. Sensitivity: Nonradioactive Probes Have Lower Sensitivity Compared to Radioactive Probes. Signal Amplification Techniques May Be Required to Enhance the Detection Limits, Especially When Working With Low-Abundance targets.
4. Quantification: Nonradioactive Probes Can Provide Quantitative Measurements Depending on the Specific Detection Method Employed. Techniques Such as Enzyme-Linked Immunosorbent Assays (ELISA) Can Enable Quantification of Target Molecules Based on Enzymatic reactions.
5. Safety: Nonradioactive Probes Offer Improved Safety Compared to Radioactive Probes as They Eliminate the Risks Associated With Working With Radioactive Materials. This Makes Them Safer for Researchers and Minimizes Environmental concerns.
6. Versatility: Nonradioactive Probes Offer Versatility in Terms of Marker Selection and Compatibility With Various Experimental Techniques. Different Types of Nonradioactive Markers Allow for Customization and Multiplexing Experiments, Enabling the Detection of Multiple Targets simultaneously.
The Choice Between Radioactive and Nonradioactive Probes Depends on Factors Such as Sensitivity Requirements, Safety Considerations, Experimental Design, and the Specific Goals of the Research or Diagnostic Application. Researchers Should Carefully Evaluate These Factors and Select the Most Suitable Probe Type for Their Specific needs.
Conclusion
Both Radioactive and Nonradioactive Probes Are Widely Used in Molecular Biology for the Detection and Analysis of DNA, RNA, and Proteins. Radioactive Probes Have High Sensitivity but Also Pose Safety Concerns and Require Special Handling and Disposal Procedures.
Nonradioactive Probes Are Safer and More Stable but May Be Less Sensitive and Produce Higher Background Noise. the Choice of Probe Depends on the Specific Application and the Researcher’s Preferences and Safety considerations. If You Want to Learn More About the Difference Between Radioactive and Nonradioactive Probes, You Can Consult Scientific Literature or Consult With a Molecular Biology Expert.