Hydrolysis, the Process of Breaking Complex Molecules Down by Adding Water, Plays an Integral Part in Many Industries Such as Food Processing, Pharmaceuticals, and Biofuel Manufacturing.
Acid hydrolysis and the enzymatic method are two widely employed hydrolytic processes that aim for similar results regarding substances used and conditions required for their implementation. We will explore their differences, their mechanism applications advantages, and drawbacks further in this article.
Definition of Acid Hydrolysis and Enzymatic Hydrolysis
Acid Hydrolysis: Acid hydrolysis is the process of dismantling chemical or molecular bonds by adding water molecules in an acidic environment, with acid catalysts helping break them. An acid releases a proton that leads to the formation of hydronium Ions (H3O+). Acid hydrolysis has wide applications both industrially and laboratory-wise for breaking apart organic and inorganic substances.
Enzymatic Hydrolysis: Enzymatic Hydrolysis Refers to the Process of Breaking Apart Complex Molecules Using Specific Enzymes. Enzymes are biological catalysts that facilitate chemical reactions by lowering the activation energy required for the reaction to occur. Compounds containing water molecules are enhanced through enzyme hydrolysis by adding specific enzymes that serve as catalysts, attaching themselves to the substrate and breaking between covalent bonds. Enzyme hydrolysis plays a central role in biochemical processes like digestion where enzymes play their part by breaking food down into smaller particles that the body can easily assimilate; It has many applications across industries including producing biofuels or pharmaceuticals.
Importance of hydrolysis in biological processes
Hydrolysis Plays an Essential Part in Many Biological Processes.
Here are some key reasons why hydrolysis is important in biological systems:
1. Digestion: Hydrolysis Is Essential for the Breakdown of Complex Food Components Into Smaller, More Easily Absorbable Molecules. Enzymes Play an Essential Role in Hydrolysis Reactions That Break Down Proteins, Carbohydrates, and Lipids Into Their Constituent Parts Like Amino Acids or Fatty Acids and Vice Versa.
2. Energy release: Hydrolysis reactions are involved in the release of energy from various biomolecules. Hydrolysis of Adenosine Triphosphate (ATP) Into Adenosine Diphosphate (ADP), Coupled With Inorganic Phosphate (Pi), Produces the Energy Necessary for Cell Activities and Drive.
3. Cellular metabolism: Hydrolysis reactions are central to cellular metabolism. These Enzymes Play an Essential Part in Breaking Down Complex Molecules Such as Glycogen and Triglycerides Into Their Constituent Parts, Producing Energy for Cell Processes While Maintaining Homeostasis and Metabolic Equilibrium.
4. Synthesis of biomolecules: Hydrolysis reactions are also crucial in the synthesis of biomolecules. Protein synthesis occurs by breaking apart aminoacyl-tRNA molecules with high energy, liberating amino acids that can then be integrated into polypeptide chains that form.
5. Recycling and waste management: Hydrolysis is involved in the breakdown of cellular waste, including damaged organelles and macromolecules, into their constituent parts. This enables the recycling of components for cellular repair and the removal of waste materials.
6. Signaling and regulation: Hydrolysis reactions are implicated in various signaling pathways and regulatory processes. Hydrolysis of GTP to GDP, one of the key steps involved in G protein-coupled signal transduction and intracellular transmission pathways, plays an essential part in controlling their effectiveness and the transmission of information within cells.
7. Drug metabolism: Hydrolysis reactions play an integral part in drug metabolism, where our bodies’ enzymes help accelerate this process and facilitate drug breakdown and elimination from our systems.
Hydrolysis is one of the fundamental processes in biological systems, providing essential functions like the dismantling and reconstruction of biomolecules as well as energy release for waste disposal while upholding cell functions and maintaining their functionality.
What is Acid Hydrolysis?
Acid hydrolysis refers to a process wherein substances or molecules are broken apart with acidic solvents like sulfuric or hydrochloric acids, leading to their dissolution by adding water molecules directly. Covalent bonds between molecules break off, leading to acid hydrolysis occurring.
The acid used in acid hydrolysis catalyzes by donating protons (H+) to the compound, leading to the formation of hydronium ions (H3O+). Hydronium ions serve as catalysts, aiding hydrolysis by encouraging the breaking of bonds as well as creating new ones with water molecules.
Acid hydrolysis is commonly employed in various chemical and industrial processes. Chemical Processes Like Hydrolysis Are Used for Breaking Down Organic Substances Such as Esters, Carbohydrates, and Proteins. The hydrolysis of esters yields the corresponding alcohol and carboxylic acid. Carbs Like Polysaccharides May Be Hydrolyzed Into Monosaccharide Units for Hydrolysis; Proteins Can Also Be Broken Down to Their Constituent Amino Acids for Hydrolysis. Acid hydrolysis is also utilized in the laboratory for the analysis and characterization of various compounds.

Acid Hydrolysis Is an Extremely Challenging Process That Requires High Temperatures and Acid Concentrations to Work Successfully. This can limit its applicability to certain compounds and may lead to side reactions or degradation of the desired products. Enzymatic hydrolysis using specific enzymes as catalysts is an excellent choice due to its less cumbersome reaction conditions and greater selectivity.
Role of acid as a catalyst
The acid plays a crucial role as a catalyst in facilitating the hydrolysis reaction. The acid acts as a proton donor, supplying protons (H+) to the compound being hydrolyzed. This protonation leads to the formation of hydronium ions (H3O+) in the reaction medium. Hydronium ions accelerate covalent bond breakage while adding water molecules into compounds.
The role of acid as a catalyst in acid hydrolysis can be understood through the following steps:
1. Protonation: The acid donates protons (H+) to the compound, resulting in the protonation of functional groups within the compound. This protonation makes the functional groups more susceptible to nucleophilic attack by water molecules.
2. Activation of nucleophiles: The presence of the acid and the resulting hydronium ions activate water molecules as nucleophiles. The water molecules become more reactive and are better able to attack the protonated sites within the compound.
3. Cleavage of bonds: The nucleophilic attack of water molecules on the protonated sites leads to the breaking of covalent bonds within the compound. The bonds are hydrolyzed, resulting in the formation of smaller molecules or their respective functional groups.
4. Regeneration of acid: At the end of the reaction, the acid catalyst is typically regenerated. It can undergo proton transfer reactions with water molecules or other reactants, returning to its original form and ready to participate in further catalytic cycles.
The acid catalyst increases the rate of the hydrolysis reaction by lowering the activation energy required for the bond cleavage. It provides an alternative reaction pathway with a lower energy barrier, enabling the hydrolysis to occur under milder conditions than would be required in the absence of a catalyst.
Considerations should be given when selecting an acid catalyst based on both the target compound to be hydrolyzed and the reaction conditions. Different acids offer various strengths and reactivity levels when choosing their appropriate acid catalyst, so selecting one could make all of the difference in how effective and efficient hydrolysis is conducted.
Conditions required for acid hydrolysis
Several conditions are typically required for acid hydrolysis to occur effectively. These conditions aim at creating an environment favorable for hydrolysis reaction as well as increasing its efficiency to maximize the success of this procedure.
The key conditions for acid hydrolysis include:
1. Acid concentration: A sufficient concentration of acid is necessary to promote the hydrolysis reaction. Strong Acids Such as Sulfuric Acid (H2SO4) or Hydrochloric Acid (HCl) Are Often Utilized for Acid Hydrolysis Processes. The concentration of the acid will depend on the specific compound being hydrolyzed and the desired reaction rate.
2. Temperature: Acid hydrolysis reactions are typically carried out at elevated temperatures to accelerate the reaction kinetics. Higher temperatures increase the molecular movement and collision frequency, facilitating the interaction between the acid, water, and the compound being hydrolyzed. However, excessively high temperatures should be avoided to prevent the decomposition or degradation of the desired product.
3. Reaction time: Sufficient reaction time is required for the acid hydrolysis reaction to reach completion. The time required for reactions is dependent upon factors like the chemical nature and concentration of acid as well as the desired level of hydrolysis. Longer reaction times may be necessary for more complex compounds or when working with lower acid concentrations.
4. Water content: Adequate water availability is essential for the hydrolysis reaction to take place. The addition of water, along with the acid, provides the necessary medium for the cleavage of covalent bonds. Although the Substance Being Hydrolyzed Might Already Contain Water Molecules, in Certain Circumstances Additional Amounts Might Need to Be Added to Achieve Complete Hydrolysis.
5. pH control: Monitoring and controlling the pH of the reaction mixture is important for optimizing the acid hydrolysis process. The pH influences the activity and stability of the acid catalyst and can affect the selectivity and efficiency of the hydrolysis reaction. pH adjustments can be made by adding acid or base as needed during the reaction.
6. Stirring or agitation: Stirring or agitation of the reaction mixture helps in maintaining homogeneity, facilitating mass transfer, and ensuring uniform contact between the acid, water, and the compound being hydrolyzed. This promotes efficient hydrolysis and prevents localized concentration gradients within the reaction mixture.
Keep in mind that acid hydrolysis conditions will vary based on both the compound being hydrolyzed and desired results. Optimizing these conditions through experimentation and understanding the properties of the compound are key to achieving effective acid hydrolysis.
What is Enzymatic Hydrolysis?
Enzymatic hydrolysis is a process that involves the breakdown of complex compounds or molecules into simpler components using specific enzymes as catalysts. Enzymes are biomolecules composed of proteins, which serve as highly efficient catalysts during biochemical reactions.
Water molecules are added to the compound in the presence of a specific enzyme. The enzyme facilitates the hydrolysis reaction by binding to the substrate (the compound being hydrolyzed) at its active site. This binding brings the substrate and water molecules into proximity, allowing for the precise and selective cleavage of specific bonds within the substrate.
Enzymes are very specific in their recognition of substrates. Each enzyme was specifically engineered to interact with certain compounds or groups of substances as substrates for functioning properly. Proteases catalyze the hydrolysis process for proteins; lipases aid cholesterol hydrolysis; amylases play their part by hydrolyzing carbs into sugar.
Enzymatic hydrolysis occurs under relatively mild reaction conditions. The reaction typically takes place at physiological temperatures and pH values that are suitable for the specific enzyme involved. These conditions help preserve the structural integrity of the substrate and prevent unwanted side reactions or degradation.
Enzymatic hydrolysis is a fundamental process in various biological systems. Hydrolysis plays an integral part in digestion processes, with enzymes within the digestive system breaking down complex food into simpler molecules that are easily absorbed. Enzymatic hydrolysis also plays an essential part in cell metabolism where enzymes break down macromolecules to produce energy for use within cell processes as well as building elements needed for cell processes.
Enzymatic hydrolysis is an integral process used in food industry operations such as processing, pharmaceutical production, and biofuel development. Enzymes are used in these fields to carry out precise and selective hydrolysis reactions, allowing for efficient and sustainable synthesis and degradation of various compounds.
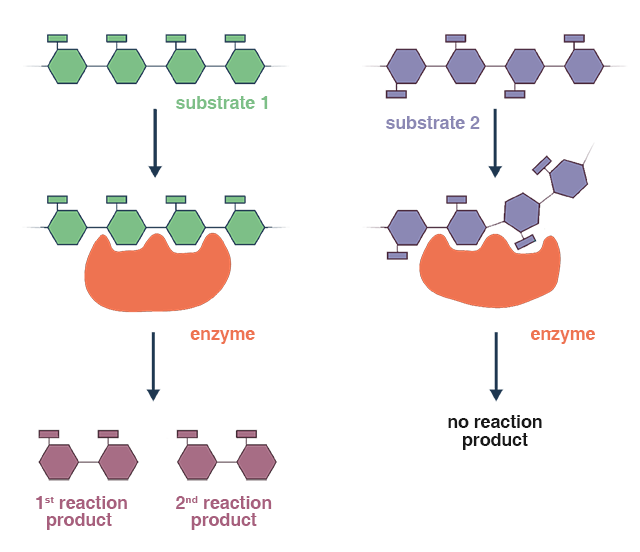
Comparable with acid hydrolysis, the enzymatic method provides many advantages; such as enhanced specificity, less reactive conditions required, and greater control of the process. Enzymatic hydrolysis may be limited by factors such as enzyme availability, cost, and stability, which need to be considered when applying this process in practical settings.
Role of Enzymes as Catalysts
Enzymes play a crucial role as catalysts in biochemical reactions. Their primary function is to speed up reactions by decreasing the energy required for them to take place.
Here are the key roles of enzymes as catalysts:
1. Lowering activation energy: Enzymes provide an alternative reaction pathway with a lower activation energy barrier. By binding to the substrate molecules at their active sites, enzymes facilitate the formation of the transition state, allowing the reaction to proceed more readily. This lowers the energy required for the reactants to reach the transition state and increases the reaction rate.
2. Specificity and selectivity: Enzymes exhibit high specificity and selectivity towards their substrates. Each enzyme is designed to recognize and bind to a specific substrate or a class of substrates based on their molecular shape, charge, and functional groups. This specificity ensures that enzymes catalyze specific reactions with high precision and efficiency while excluding other molecules.
3. Substrate binding and orientation: Enzymes play a crucial role in bringing the substrate molecules together in the correct orientation for the reaction to occur. The active site of an enzyme is complementary to the shape and properties of the substrate, allowing for specific and tight binding. This brings the reactive groups of the substrate in proximity to each other, enhancing the likelihood of bond rearrangements and facilitating the catalytic reaction.
4. Transition state stabilization: Enzymes stabilize the transition state of the reaction, which is the high-energy intermediate state that forms during the reaction. The active site of the enzyme provides an environment that allows the transition state to be more stable than the substrate or product states. This stabilization lowers the energy barrier further and promotes the efficient conversion of substrates to products.
5. Catalytic groups and cofactors: Enzymes often contain catalytic groups or cofactors that actively participate in the catalytic process. These groups can donate or accept protons, electrons, or functional groups, facilitating the necessary chemical transformations. For example, cofactors like metal ions or coenzymes can serve as electron carriers or provide essential functional groups for catalysis.
6. Regulation of reaction rate: Enzymes can be regulated to control the rate of reactions in response to cellular needs. Temperature, pH levels, and certain compounds (activators and inhibitors) all influence enzyme activity for precise regulation of metabolic pathways and biochemical processes.
Enzymes as catalysts are highly efficient and specific, and enable the remarkable complexity and efficiency of biochemical reactions in living organisms. Every aspect of our daily lives relies on these proteins for essential biological processes like metabolism and DNA replication, signal transduction, and other essential processes.
Types of enzymes involved in hydrolysis reactions
Hydrolysis reactions involve numerous enzymes, each designed specifically for breaking down specific substances.
Here are a few popular classes of enzymes involved with this process:
1. Proteases: Proteases, also known as peptidases or proteolytic enzymes, catalyze the hydrolysis of peptide bonds in proteins. They break down proteins into smaller peptides and amino acids. Examples of proteases include pepsin, trypsin, and chymotrypsin.
2. Lipases: Lipases catalyze the hydrolysis of ester bonds in lipids, such as triglycerides, phospholipids, and sterol esters. They break down these complex molecules into fatty acids and glycerol. Lipases are involved in processes like fat digestion and lipid metabolism. Examples of lipases include pancreatic lipase and gastric lipase.
3. Amylases: Amylases are enzymes that hydrolyze starch and glycogen, which are complex carbohydrates, into smaller sugar units like glucose and maltose. They break down the α-1,4-glycosidic bonds in these polysaccharides. Salivary amylase and pancreatic amylase are examples of amylases.
4. Cellulases: Cellulases are enzymes that hydrolyze cellulose, a complex carbohydrate found in plant cell walls, into glucose. They break down the β-1,4-glycosidic bonds in cellulose. Cellulases are produced by microorganisms like bacteria and fungi, as well as certain animals like termites.
5. Nucleases: Nucleases are enzymes involved in the hydrolysis of nucleic acids, such as DNA and RNA. They break down the phosphodiester bonds between nucleotides, resulting in the release of nucleotide monomers. Examples include DNAse and RNAse.
6. Glycosidases: Glycosidases catalyze the hydrolysis of glycosidic bonds in carbohydrates and glycoconjugates. They are involved in the breakdown of complex carbohydrates like disaccharides, oligosaccharides, and glycoproteins. Examples include lactase, sucrase, and β-glucosidase.
7. Esterases: Esterases hydrolyze ester bonds, which are found in a wide range of compounds including lipids, carbohydrates, and certain drugs. They break down esters into their corresponding alcohol and carboxylic acid components. Examples of esterases include acetylcholinesterase and lipoprotein lipase.
These are just a few examples of the various types of enzymes involved in hydrolysis reactions. Each enzyme is specialized to catalyze the hydrolysis of specific types of compounds, ensuring the precise breakdown of complex molecules into simpler components.
Proteases for protein hydrolysis
Proteases are enzymes specifically involved in the hydrolysis of peptide bonds in proteins. They break down proteins into smaller peptides and amino acids. Proteases play essential roles in various biological processes, including protein digestion, cellular protein turnover, and protein modification.
Here are some common proteases used for protein hydrolysis:
1. Trypsin: Trypsin is a serine protease produced in the pancreas and released into the small intestine. It cleaves peptide bonds on the carboxyl side of basic amino acids such as lysine and arginine. Trypsin is commonly used in protein digestion experiments and protein sequencing methods.
2. Chymotrypsin: Chymotrypsin is a serine protease enzyme that disrupts carboxyl-end peptide bonds on amino acids such as Tyrosine, Phenylalanine, and Tryptophan. It is involved in the digestion of dietary proteins in the small intestine.
3. Papain: Papain is a cysteine protease obtained from papaya fruit. It has broad substrate specificity and can hydrolyze peptide bonds at various amino acid residues. Papain is widely used in biochemical research, protein purification, and as a meat tenderizer.
4. Bromelain: Bromelain is a mixture of proteolytic enzymes derived from pineapple stems. It contains various proteases, including cysteine proteases and serine proteases. Bromelain is used for protein digestion, meat tenderization, and as a dietary supplement.
5. Thermolysin: Thermolysin is a metalloprotease that requires metal ions like zinc for its activity. It cleaves peptide bonds on the carboxyl side of hydrophobic amino acids such as leucine, isoleucine, and valine. Thermolysin is often used in protein crystallization and peptide mapping studies.
6. Pepsin: Pepsin is a gastric protease produced in the stomach. It has optimal activity in acidic conditions and cleaves peptide bonds on the carboxyl side of aromatic amino acids. Pepsin plays a crucial role in the initial stages of protein digestion in the stomach.
7. Subtilisin: Subtilisin is a serine protease produced by bacteria, such as Bacillus subtilis. It has broad substrate specificity and can cleave peptide bonds at various amino acid residues. Subtilisin is commonly used in industrial applications, such as laundry detergents and the synthesis of peptides.
These are just a few examples of proteases used for protein hydrolysis. Each protease has its specificity and optimal reaction conditions, making it suitable for different applications in research, industry, and medicine.
Comparison Chart
Sure! Here’s a comparison chart highlighting the key differences between acid hydrolysis and enzymatic hydrolysis:
Aspect | Acid Hydrolysis | Enzymatic Hydrolysis |
---|---|---|
Catalyst | Acid | Enzymes |
Reaction Specificity | Non-specific | Highly specific |
Reaction Conditions | High temperatures and strong acid concentrations | Milder conditions resembling physiological environments |
Selectivity | Lack of selectivity | High selectivity |
Biocompatibility | Not biocompatible | Biocompatible |
Substrate Range | A broad range of compounds | Specific compounds or classes of compounds |
Product Stability | This may result in the degradation of compounds | Preserves the integrity of compounds |
Reaction Control | Limited control over the reaction | Controlled and targeted hydrolysis |
Applications | Industrial processes, degradation of compounds | Biological research, food processing, pharmaceutical production, etc. |
Please note that this chart provides a general comparison and that there may be specific variations or exceptions depending on the compounds, acids, or enzymes used in each hydrolysis method.
Conclusion
Acid hydrolysis and enzymatic hydrolysis are two distinct methods of breaking down complex molecules into smaller components. Acid hydrolysis relies on strong acids as catalysts, offering high efficiency and versatility in various applications. Enzymatic hydrolysis, on the other hand, utilizes enzymes to selectively target specific substrates, providing higher purity, milder reaction conditions, and reduced environmental impact.
Understanding the differences between acid hydrolysis and enzymatic hydrolysis allows for informed decision-making in choosing the appropriate method for specific industrial processes, ultimately contributing to the advancement of various fields.